Noble-Metal Nanostructures with Controlled Morphologies
Majiong Jiang1, Claire M. Cobley2, Dr. Byungkwon Lim2, Prof. Younan Xia2
1Department of Chemistry, 2Washington University, St. Louis, MO
Introduction
Noble-metal nanostructures are widely used in a variety of applications ranging from catalysis to electronics, surface plasmon resonance (SPR), surface-enhanced Raman scattering (SERS), and biomedical research.1-3 Different applications demand nanostructures with specific morphologies. For example, catalysis often requires the noble-metal nanostructures to be dispersed on ceramic supports with high surface areas, while nanowires are needed for transport measurements and as interconnects in electronic devices. Morphology may also provide a sensitive parameter for enhancing and/or tailoring the properties of noblemetal nanostructures. It has been established that the catalytic activity and selectivity of noble-metal nanoparticles are strongly dependent on the facets exposed on the surface. For Pt, its activity for the oxygen reduction reaction (ORR) increases in the order of (100) < (111) < (110) in a non-adsorbing electrolyte.4 In the case of Pd, its (100) facets are almost four times more reactive than the (111) facets for formic acid oxidation.5 On the other hand, the morphology of Au or Ag nanostructures not only determine SPR characteristics, but also the merits in SERS detection.6 Therefore, controlling the morphologies of noble-metal nanostructures is crucial to enhancing their performance in various applications.
Polyol Synthesis of Nanostructures
The polyol process has become a popular method for preparing noble-metal nanostructures with well-defined morphologies. The primary step in this process involves heating a polyol such as ethylene glycol (EG) (Product No. 324558) with a metal salt (i.e., a precursor to the noble metal) in the presence of a polymeric capping agent such as poly(vinylpyrrolidone) (PVP) (Product No. 234257, 856568, 437190) to generate metal atoms. Upon heating EG to the temperature range of 140-160 oC, glycolaldehyde (Product No. G6805) (the reducing agent) is produced through the following oxidation reaction:7
2HOCH2CH2OH + O2 → 2HOCH2CHO + 2H2O
The ability of polyols to dissolve many metal salts and their temperature-dependent reducing power makes polyol synthesis an attractive route to nanostructures of various noble metals including Ag, Au, Pd, Pt, Rh, Ru, and Ir.
Control of Nanostructure Morphology
In solution-phase synthesis of noble-metal nanostructures, the final morphology is primarily determined by the twin structure of seeds and the growth rates of different crystallographic facets. In the early stage of a reaction, a precursor compound is either decomposed or reduced to generate zero-valent atoms, which can form a cluster with fluctuating structure also known as a nucleus. Once the cluster has grown past a critical size, structural fluctuations become so energetically costly that it will be locked into a well-defined structure and become a seed. As illustrated in Figure 1, the seed serves as a bridge between atoms and the nanostructure, and it may take a single-crystal or multiply twinned structure.8 In practice, the twin structure of seeds can be controlled by manipulating the reduction kinetics. When the reduction rate is relatively fast, thermodynamically favorable species such as single-crystal and multiply twinned seeds are dominant in the nucleation stage. The former can evolve into octahedrons or cubes, while the latter into decahedrons or pentagonal nanowires depending on the reaction conditions. When the reduction rate is considerably slowed down, the reaction will become kinetically controlled. In this case, platelike seeds with planar defects such as stacking faults can form at the initial nucleation stage, which then evolve into hexagonal and triangular nanoplates that deviate from the thermodynamically favored morphologies. The distribution of differently twinned seeds can be further altered by selectively employing or blocking oxidative etching, in which zero-valent metal atoms are oxidized back to ions. In one case, the twinned seeds can be selectively removed by the O2/Cl- etchant, leaving behind single-crystal seeds in the reaction solution.9 In most cases, the introduction of selective capping agents provides a powerful means of controlling the relative growth rates of different crystallographic facets as they can interact strongly with specific facets and thus change the relative free energies for different facets. This kind of chemisorption or surface capping has a profound impact on the final morphology of metal nanostructures. For instance, PVP is a polymeric capping agent whose oxygen atoms bind most strongly to the {100} facets of Ag and thus facilitate the formation of Ag nanowires or nanocubes.10,11
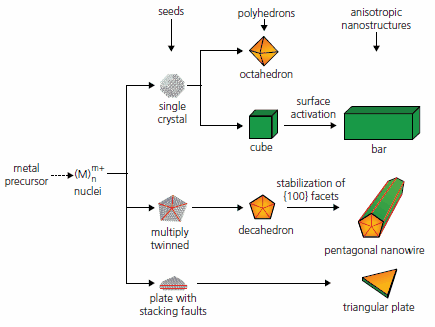
Figure 1.Schematic illustration of the reaction pathways that lead to face-centered cubic metal nanostructures with different morphologies. First, a precursor is reduced or decomposed to form the nuclei (small clusters). Once the nuclei have grown past a certain size, they become seeds with a single-crystal or multiply twinned structure. If stacking faults are introduced, plate like seeds will be formed. The green and orange colors represent the {100} and {111} facets, respectively. Twin planes are depicted with red lines.8
Case Study I: Silver (Ag)
Silver nanostructures are probably best known for their morphology-dependent optical properties like SPR.11 They also serve as the most popular substrates for SERS and the most selective catalyst for epoxidation of ethylene. Uniform Ag nanowires can be produced by adding CuCl2 (Product No. 203149) to a typical polyol reduction of AgNO3 (Product No. 204390) with EG (Figure 2a).12 The Cu(II) ions will be reduced by EG into Cu(I). Both the Cu(I) and Cl- ions play important roles. Copper ions promote the growth of wires by reducing the amount of oxygen on the surface, preventing the multiply twinned seeds from being dissolved due to oxidative etching and unblocking sites for further deposition of Ag atoms. Ethylene glycol can recycle Cu(II) back to Cu(I), so only a small amount of Cu(II) ions needs to be added to scavenge the adsorbed oxygen on the metal surface. Chloride ions control the concentration of free Ag+ in the solution by forming AgCl, consequently decreasing the reduction rate and facilitating preferential growth on the high energy twin boundaries at the ends of the wire. In a typical synthesis, PVP is also required to preferentially passivate the {100} surfaces that make up the sides of the nanowires.
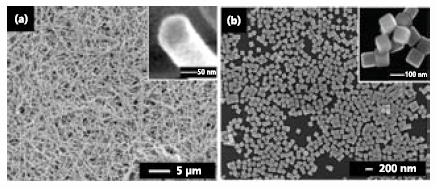
Figure 2.Silver nanostructures synthesized by the modified polyol process. (a) SEM image of Ag nanowires synthesized by the addition of CuCl2. Inset shows the pentagonal cross section of the nanowires. Reprinted from Ref. 12 with permission from the Royal Society of Chemistry. (b) SEM image of Ag nanocubes synthesized by the addition of NaHS.27
Trace amounts of other ionic species are also important in the morphological control of Ag nanostructures. By adding small amounts of sulfide in the form of either Na2S (Product No. 407410) or NaHS (Product No. 161527) to the polyol reduction of AgNO3, Ag nanocubes can be routinely produced as uniform samples and in relatively large quantities (Figure 2b).13 Since Ag2S is a known catalyst for Ag reduction, the proposed mechanism involves small Ag2S seeds generated immediately after the addition of AgNO3. These seeds catalyze the reduction and serve as sites for the growth of high quality cubes. Since the reaction is kinetically controlled, more thermodynamically favorable twinned seeds are not able to form, and the final product is dominated by single-crystal species like nanocubes. PVP again plays the role of preferentially capping the {100} faces of Ag nanocubes.
Case Study II: Gold (Au)
Gold nanostructures have received considerable attention due to their excellent chemical stability, bio-inertness, SPR/SERS properties, and unique catalytic activity. There have been many demonstrated methods for controlling the morphology of Au nanostructures.14-16 As for the Ag system, the selection of reducing agent, stabilizer, and reaction temperature is critical to forming a particular morphology. In most cases, strong reducing agents, as exemplified by polyol reduction, facilitate the formation of thermodynamically favored polyhedral Au nanostructures. There are, however, notable differences. For example, in contrast to the Ag system, the binding of PVP to Au does not appear to be sufficiently strong to promote the formation of {100} facets. As a result, single-crystal and multiply twinned polyhedrons enclosed by {111} facets such as octahedrons, icosahedrons, and decahedrons are dominant morphologies for Au nanostructures in the polyol synthesis.
For instance, Au octahedrons have been synthesized in high yields via a modified polyol process by using polyethylene glycol 600 (Product No. 202401) as a reducing agent, which also serves as a solvent in the synthesis (Figure 3a).17 In this case, the addition of a small amount of sodium borohydride (NaBH4) (Product No. 480886), prior to the addition of an aqueous gold(III) chloride (AuCl3) solution (Product No. 334049), is the key to high-yield production of uniform Au octahedrons. It serves as a strong reducing agent and thus ensures the fast reduction of the Au precursor. On the other hand, the multiply twinned nanostructures of Au such as decahedrons can be produced at a relatively high concentration of PVP in the polyol reduction of HAuCl4 (Product No. 484385) with diethylene glycol (Product No. H26456) (Figure 3b).18 It seems that the high concentration of PVP helps to block the surfaces of Au decahedrons from the oxidative etching by O2/Cl- pair. As a result, these twinned nanostructures could be stabilized and accumulate throughout the reaction.
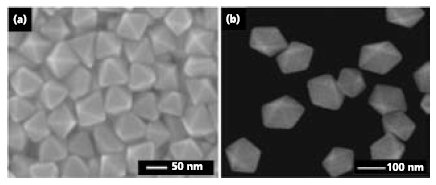
Figure 3.Gold nanostructures synthesized by the modified polyol process. (a) SEM image of Au octahedrons made by the addition of a small amount of NaBH4 in a polyol reduction of AuCl3 with polyethylene glycol 600. Reprinted from Ref. 17 with permission from Wiley-VCH. (b) SEM image of Au decahedrons obtained by a polyol reduction of HAuCl4 with diethylene glycol in the presence of a high concentration of PVP.18
Case Study III: Palladium (Pd)
Palladium is a key catalyst for a variety of reactions including hydrogenation, dehydrogenation, and carbon-carbon bondforming processes such as Suzuki, Heck, or Stille coupling. In general, the predominant morphology of Pd nanostructures in a typical polyol reduction of Na2PdCl4 (Product No. 379808) with EG is the truncated octahedron.19 Morphological control of Pd nanostructures can be achieved by introducing specific capping agents such as Br- ions and/or by employing oxidative etching. In one example, Pd nanobars with a rectangular crosssection can be synthesized by adding Br- ions to the polyol reduction of Na2PdCl4.20 Bromide ions bind strongly to the {100} facets of Pd, resulting in the formation of cubic Pd seeds in the nucleation stage. The formation of Pd nanobars can be attributed to anisotropic growth of the cubic seeds driven by a selective activation process, which involves the localized oxidative etching on one of the six faces of the cubic seed to remove some of the Br- ions. This facilitates preferential growth of the cubic seed along one direction and eventually leads to the formation of Pd nanobars enclosed by the {100} facets (Figure 4a).
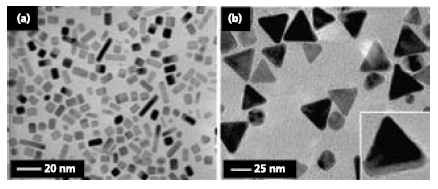
Figure 4.Palladium nanostructures synthesized by the modified polyol process. (a) TEM image of Pd nanobars synthesized by the addition of KBr in a polyol reduction of Na2PdCl4 with EG in the presence of a small amount of water as a co-solvent. Reprinted from Ref. 20 with permission from the American Chemical Society. (b) TEM image of triangular Pd nanoplates synthesized by introducing a small amount of FeCl3 and HCl into a polyol reduction of Na2PdCl4 with EG.21
As another demonstration, Pd triangular nanoplates have been synthesized by introducing Fe(III) species into the polyol synthesis (Figure 4b).21 The reduction rate is substantially slowed down due to the presence of two wet etchants for Pd(0), i.e., Fe(III) species and the O2/Cl- pair. The oxidative etching can be further enhanced by adding an acid to lower the pH of the reaction solution. This kinetically controlled process under the slow reduction rate enables the formation of plate-like seeds in the nucleation stage, which then gradually evolve into triangular nanoplates via preferential addition of newly formed Pd atoms to the side faces of developing nanoplates. These nanoplates of Pd exhibit SPR peaks in the visible region due to their increased sizes. Thanks to the sharp corners and edges, they could serve as active substrates for SERS.
Case Study IV: Platinum (Pt)
Platinum is an invaluable catalyst for a variety of industrial applications, such as hydrogenation, oil cracking, and CO/NOx oxidation in catalytic converters. It also serves as the most effective electrocatalyst for both ORR and fuel oxidation in the operation of a fuel cell.22,23 For most of these applications, it is critical to disperse Pt nanostructures on solid supports. The polyol reduction can be modified to grow Pt nanowires on various substrates by adding a small amount of Fe(II) or Fe(III). In a typical process, Pt(II) species are formed by reducing H2PtCl6 (Product No. 262587) with EG at 110 oC in the presence of PVP. By adding a trace amount of FeCl3 (Product No. 451649) as an oxidative etchant for Pt(0), the nucleation of Pt atoms can be controlled to grow into uniform nanowires directly on a Pt or W gauze (Figure 5a).23 The Pt gauze (Product No. 298107, 298093) covered with Pt nanowires showed enhanced activity, over 1.5 times higher than that of the Pt/C (E-TEK) catalyst, towards the methanol oxidation reaction. In addition, Pt nanowires can also be readily grown on other substrates such as electrospun TiO2 nanofibers and patterned silicon substrates using this simple approach.24,25
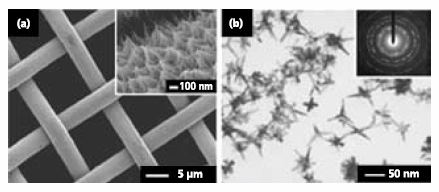
Figure 5.Platinum nanostructures synthesized by the modified polyol process. (a) SEM image of Pt nanowires on Pt gauze. Inset shows the edge view of the Pt nanowires growing outward from the surface of the gauze. Reprinted from Ref. 23 with permission from the American Chemical Society. (b) TEM image of Pt multipods synthesized through an iron-mediated polyol process. In this case, the kinetics of polyol reduction is controlled by abruptly blocking oxidative etching with N2 flow, which initiates overgrowth of Pt seeds into highly branched nanostructures.26
In the absence of substrates, highly branched nanostructures of Pt can be produced through a similar mechanism.26 The key to the formation of branched nanostructures is to abruptly block oxidative etching by flowing N2 (Product No. 295574) and thus removing O2 in the reaction system. In this case, the concentration of Pt atoms dramatically increases to a high level, leading to overgrowth of the seeds into highly branched nanostructures such as multipods (Figure 5b).
Conclusion
We have demonstrated that synthesis of noble-metal nanostructures with various morphologies can be achieved by the polyol method. Although the exact mechanisms related to the formation of particular morphologies are yet to be fully understood, it has been proven that some of the important reaction parameters such as reduction kinetics, oxidative etching, and surface capping could be combined to provide an effective route to maneuver both the twin structure of seeds and the facets of the seed surface, the two key factors in determining the final morphology of the nobel-metal nanostructure. Further improvement in this research area will be possible as our understanding of the nucleation and growth mechanisms is advanced. The ability to control the morphology of noble-metal nanostructures provides a great opportunity to realize a variety of fascinating morphology-dictated properties and applications.
References
如要继续阅读,请登录或创建帐户。
暂无帐户?