Fouling Resistant PEG Based Grafted Polymer Coatings
Lesley M. Hamming, Prof. Phillip B. Messersmith
Biomedical Engineering Department Northwestern University Evanston, IL
Material Matters 2008, 3.3, 52.
Accumulation of biological matter at surfaces is an inevitable event in virtually any environment in which natural and man-made materials are used. Although sometimes fouling of surfaces with biomolecules and bioorganisms has little consequence, biofouling must be minimized or controlled in order to maintain performance and safety of devices and structures. For example, in the medical environment, components of biofluids such as proteins, cells and pathogens have a propensity to strongly adhere to surfaces, altering performance with potentially hazardous outcomes. Urinary tract infections resulting from microbial colonization of catheters represents the most common hospital-acquired infection.1 Implantable medical devices are also susceptible to microbially influenced corrosion (MIC) leading to the need for replacement surgeries with increased risk of infection.2 Additional examples of surfaces prone to biofouling include the hulls of marine vessels which often become coated with marine organisms and their secretions, decreasing the efficiency of propulsion and contributing to higher fuel consumption.3 This article will briefly highlight approaches for limiting biofouling of surfaces using grafted polymers, including biomimetic strategies for linking polymers onto surfaces.
A common strategy for preventing biofouling is to graft an anti-fouling polymer onto a surface as depicted in Figure 1.4 Key features of such grafted polymer systems include chemical characteristics, molecular weight and architecture of the antifouling polymer, and the method by which the polymer is grafted to the surface. One of the most extensively studied anti-fouling polymers is poly(ethylene glycol) (PEG), a water soluble polymer with low toxicity and extensive history of use in medicine and drug delivery.5 PEG is widely available to academic and industrial researchers through either direct synthesis or purchase, and with appropriate chemical derivatization can be grafted onto surfaces to reduce the nonspecific adsorption of proteins, cells and bacteria (see Table on p. 54 of this issue). Although the thermodynamic and molecular mechanisms for the protein and cell resistance of surface immobilized PEG are not completely understood, numerous studies have determined that steric hindrance effects, chain length, grafting density, chain conformation, and hydrophilicity of the grafted polymer play important roles in resisting protein adhesion.6-8

Figure 1.Grafted antifouling polymer systems consist of an antifouling polymer (blue lines) anchored by specific functional groups (triangles) onto a substrate (gray), providing a physical barrier to adsorption of proteins (red), cells (blue) and bacteria (green) onto the underlying surface.
Grafting of antifouling polymers onto surfaces can be accomplished using two basic strategies. The graft-to approach consists of surface adsorption of pre-synthesized polymer functionalized with a chemical anchoring group. In contrast, graft-from approaches rely on in-situ polymerization of polymer from a grafted initiator. Graftto systems are typically of monolayer thickness (a few nanometers), and can be formed through physisorption or chemisorption of polymer from a solvent. Graft-from systems are generally much thicker (10–100 nanometers or more) but require pre-functionalization of the substrate with a suitable initiator. Essential to both approaches, however, is the ultimate requirement of either physisorptive or chemisorptive interactions to hold the polymer onto the surface. Physisorption relies on relatively weak Van der Waals or hydrophobic forces to tether polymers onto a surface, with an example being the adsorption of Pluronic-type block copolymers onto hydrophobic substrates.9 Chemisorption typically provides more robust linking of polymer to substrate, and is exemplified by gold-thiolate,10 metal oxide-silane linkages,11 and electrostatic interactions.12
It has recently become apparent that strategies employed by biological organisms can provide inspiration for new approaches to grafting polymers onto surfaces. Of particular interest are unusual amino acids found in marine adhesive proteins and used to secure robust attachment to wet surfaces. Marine mussels adhere firmly to a variety of material surfaces such as rocks, wood, animals, and shells even in a wet and turbulent environment. The amino acid 3,4-dihydroxyphenylalanine (DOPA) (D9628 ) is present at concentrations of up to 27 mol% in proteins located near the interface of the mussel’s adhesive pad and the substrate (Figure 2).13 DOPA contributes remarkable adhesive properties as demonstrated by single molecule force spectroscopy,14 forming strong chemical interactions with both organic and inorganic surfaces.

Figure 2.Biologically inspired strategies for grafting antifouling polymers onto surfaces. (a) Image of a mussel adhered to a substrate, (b) Schematic of the adhesive pad and interfacial location of mussel adhesive proteins (Mefp3, Mefp5) with the highest content of the amino acid 3,4-dihydroxyphenylalanine (DOPA), (c) Chemical structure of DOPA, (d) Example graft-to biomimetic polymer showing an adhesive peptide anchor and antifouling PEG polymer, (e) Example graft-from biomimetic polymer showing a surface bound biomimetic initiator and polymerized PEG polymer.
Chemical versatility and robustness of DOPA have recently been exploited in a number of ways to link antifouling polymers onto surfaces.15 Polymers such as monomethoxyterminated PEG conjugated with DOPA containing peptides combine the attractive antifouling features of PEG with an adhesive anchor allowing for convenient attachment to surfaces via the graft-to approach.16 Linear PEG polymers derivatized with 1–3 DOPA residues (Figure 2) were found to adsorb to titanium oxide (TiO2) and gold substrates,17 and conferred high resistance to serum components as measured by optical waveguide spectroscopy (OWLS) and spectroscopic ellipsometry (ELM). In-situ adsorption of serum revealed that the TiO2 control surface accumulated 250 ng/cm2 of serum protein while the surfaces modified with mPEG-DOPA3 only accumulated less than 1 ng/cm2 confirming the excellent nonfouling characteristics of PEG conjugated with DOPA.17 More sophisticated peptide anchors have also been developed; for example, a decapeptide analog of Mytilus edulis foot protein-1 was conjugated to PEG and used to confer resistance to cell attachment on gold surfaces (Figure 3).16 Peptide conjugated polymers are synthesized by solid phase methods in cases where an exact amino acid sequence is desired, or through polymerization of N-carboxyanhydride monomers via initiation from a monoamine functionalized polymer to create an oligomeric peptide anchor with a statistical distribution of amino acids. Recently, related catechol based adhesive anchors inspired by the biological iron chelator anachelin have been used in a similar way,18 further expanding the chemical diversity of anchoring groups available for attaching PEG onto surfaces.
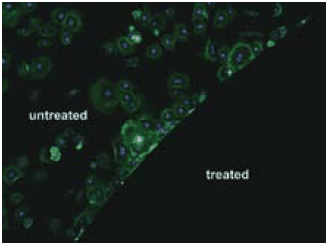
Figure 3. Fibroblast cell attachment to untreated gold (upper left) and gold grafted with PEG derivatized with a mussel adhesive protein analog decapaptide Ala-Lys-Pro-Ser-Tyr-Hyp-Hyp-Thr-DOPA-Lys (lower right).
The graft-from approach has also been employed to create antifouling coatings through polymerization of PEG macromonomers from surface-bound initiators. For example, atom transfer radical polymerization (ATRP) has been employed to polymerize acrylate-functionalized PEG from a gold surface modified with an initiator functionalized selfassembled monolayer (SAM).19 The resulting grafted polymer coating, like most other graft-from coatings, was considerably thicker than graft-to coatings and exhibited excellent protein and cell fouling resistance. With respect to biomimetic anchors, a dopamine-based ATRP initiator was synthesized for preparation of antifouling coatings on metal oxides.20 In this case, the catechol functional group found in the side chain of DOPA was exploited for adsorption onto titanium oxide and stainless steel, from which polymerization of PEG macromonomers via ATRP yielded thick fouling resistant coatings.
A hybrid graft-from/graft-to approach with remarkable versatility in its ability to functionalize different materials with antifouling polymers was recently reported.21 The method involves two steps, the first relies on polymerization of dopamine hydrochloride (H8502) at alkaline pH to form a thin (50 nm or less) adherent polydopamine coating on the surface of virtually any material. The coating process utilizes a readily available reagent and can be applied to complex shaped objects via simple dip-coating. Formation of the polydopamine coating exploits reactions reminiscent to those occurring during solidification of mussel adhesive proteins and during formation of the biological pigment melanin. The resulting coating harbors latent reactivity toward nucleophiles, facilitating a second coating step involving covalent grafting of amine or thiol functionalized PEG onto the polydopamine coated surface (Figure 4). This new approach to modification of materials promises a convenient, cost-effective and versatile strategy to confer antifouling properties onto surfaces, does not require complicated surface preparation steps, and combines elements of both graft-from (Step 1) and graft-to (Step 2) approaches.

Figure 4.Facile grafting of antifouling polymers to material surfaces using a two-step approach. (a) Schematic of the surface grafting method, consisting of surface mediated polymerization of dopamine followed by graft-to of an amine or thiol functionalized PEG. Using this approach, antifouling grafted PEG coatings can be applied to many different surfaces, including inorganic and organic materials. (b) Normalized cell attachment before (black bars) and after (red bars) modification of glass, titanium, gold, silicon nitride, Teflon (PTFE), polyurethanes (PU1, PU2) and polystyrene (PS) with polydopamine and PEG-NH2.
Grafted PEG coatings have also been investigated for inhibiting attachment of marine organisms to surfaces.22-24 Several grafted polymer designs have been employed, including hyperbranched fluoropolymer-PEG composites,22 hydrophobic polymers with PEG side chains,23 and linear PEG.24 These studies have to date emphasized fouling of diatom (Navicula perminuta) and green algae (Ulva linza) species and show enhancement of antifouling performance with increasing PEG content and favorable performance compared to standard silicone fouling-release coatings. Silicone fouling-release coatings are currently used by the marine industry to facilitate hydrodynamic removal of biofouling agents but these agents are not completely effective against all marine fouling and do not work well on slow-moving vessels. With further development, PEG-based coatings may provide effective fouling performance in the marine environment.
In summary, grafted PEG coatings can provide effective resistance to fouling of surfaces by proteins, cells, bacteria and other biological organisms. Biologically inspired anchoring moieties offer convenient, versatile and robust approaches to linking PEG onto surfaces. PEG coatings can be used to control fouling of implantable medical device surfaces, contact lenses, surgical tools, biosensors, electrophoresis capillaries for bioseparations, and fouling-prone surfaces of water treatment facilities and ship hulls.
References
如要继续阅读,请登录或创建帐户。
暂无帐户?