Principal Component Analysis (PCA) Evaluation of Seven Commercial Ionic Liquid Capillary GC Columns
Reporter US Volume 33.1
The following was generated with the assistance of an outside source using Sigma-Aldrich products. Technical content was generated and provided by:
S. Rodríguez-Sáncheza, P. Galindo-Iranzob, A.C. Soriaa, M.L. Sanza, J.E. Quintanilla-Lópeza, and R. Lebrón-Aguilarb
aInstituto de Química Orgánica General (CSIC), Madrid, Spain
bInstituto de Química-Física “Rocasolano” (CSIC), Madrid, Spain
Introduction
Ionic liquids are organic salts utilized for various industrial applications. Their unique and tunable physicochemical properties are unlike any other solvent. They typically remain liquid over wide temperature ranges, and tend to exhibit low melting points, good thermal stability, negligible vapor pressure, and high viscosity. These properties also make them ideal candidates as stationary phases for gas chromatography (GC). In fact, a quick literature search reveals a great deal of development aimed at preparing columns using ionic liquid stationary phases.
While monocationic ionic liquids can be employed for industrial applications, it was discovered that dicationic and polycationic ionic liquids make suitable GC stationary phases[1]. Currently, there are seven different commercialized capillary GC columns which use ionic liquid stationary phases. Their main advantage is that they offer different separation properties compared to columns prepared with polysiloxane polymer and polyethylene glycol stationary phases. They also exhibit lower column bleed, higher thermal stability, greater resistance to damage from moisture and oxygen, and longer life time when compared to columns of similar polarity.
McReynolds and Abraham Methods
The polarity of a GC stationary phase can be estimated using the McReynolds method, in which retention indices (I) are determined for five test probes, representing different compound classes[2]. The relationships of probes to compound classes are summarized in Table 1. Each probe relates to a set of solute-stationary phase interactions. Combining the five retention indices can then be used to determine the polarity of the stationary phase. However, this approach cannot fully differentiate individual interactions since the retention of probes is not driven by a single interaction, but is most often due to several simultaneous interactions. Thus, the imprecise property of ‘polarity’ alone is probably not sufficient to characterize GC stationary phases.
In contrast, the solvation parameter model (SPM) can quantitatively evaluate the individual intermolecular interactions between a substance and the stationary phase[3]. Used for many years to characterize HPLC phases, the SPM is also applicable for characterizing GC stationary phases[4-6]. This model is described for GC by the Abraham equation:
log k = c + eE + sS + aA + bB + lL
where k is the retention factor of a solute on the stationary phase at a specific temperature; c is the model intercept; the capital letters (E, S, A, B, and L) represent the solute descriptors that are probe-specific parameters determined for many substances; and the lowercase letters (e, s, a, b, and l) are referred to as the system constants, in which all information concerning the solvation properties of the stationary phase is represented[7]. Table 2 lists the correlation between system constants and the capacity of the stationary phase for various interactions.
Experimental
In order to cover a broad range of possible solute-stationary phase interactions, 95 solutes with varied functional groups were selected to perform stationary phase characterization by the SPM. The group of columns included seven ionic liquid columns and 45 non-ionic liquid columns. The solutes selected were grouped into different mixtures, and then chromatographed isothermally at several temperatures to obtain their k values. The following were then generated:
- A table of system constants with standard deviations and goodness-of-fit statistics at each isothermal temperature
- Radar plots of system constants, which proved a useful tool to display multivariate observations in a two-dimensional chart
- A 3D plot of a principal component analysis (PCA) using data from 120 °C isothermal runs, providing a valuable overview of selectivity
Additional details of the experimental design, the table of system constants and further discussion, several radar plots and discussion, and the 3D PCA plot can be found in reference 8.
PCA Results and Discussion
PCA is a statistical procedure that rotates and transforms the original axes, each representing an original variable (system constants in our case), into new axes called principal components (PCs) that are linear combinations of the original variables and account for most of the variance in the data. PCA can reveal those variables, or combination of variables, which describe some inherent structure in the data and these may be interpreted in chemical or physicochemical terms[9].
The 3D score plot shown in Figure 1 is an update of a plot previously reported[8]. The update is the inclusion of data for three additional ionic liquid columns. As shown, the first three principal components explain 99.2% of the variance, representing the whole column selectivity space quite well. Principal components 1 and 2 take into account contributions from the a and s system constants, while principal component 3 is mainly related to the b and e system constants. Dashed lines indicate the distribution trend of polysiloxane polymer stationary phases substituted with trifluoropropyl (line 1), phenyl (line 2), and cyanopropyl (line 3) groups. There are two other clearly differentiated groups, corresponding to polyethylene glycol (cluster I) and ionic liquid (cluster II) stationary phases.
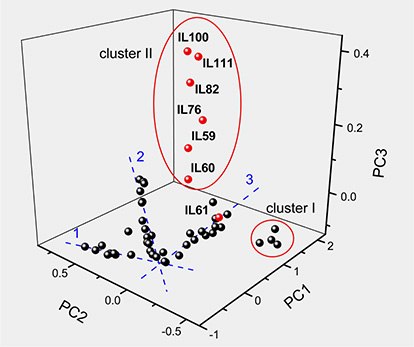
Figure 1. PCA Plot
The PCA score plot clearly reveals that most of the ionic liquid stationary phases possess separation characteristics unlike any non-ionic liquid stationary phase. They are the only ones that have high values of both PC1 and PC3. That is, among all the stationary phases characterized, only the ionic liquids are capable of simultaneously providing intense H-acceptor plus dipolar interactions (high a + s value [high PC1]) and intense H-donor plus π-π interactions (high b + e value [high PC3]).
The solvation characteristics of the SLB®-IL61 column were found to be more similar to cyanopropyl substituted polysiloxane polymer phases (line 3) than to the other ionic liquid phases (cluster II). This is due to the trifluoromethylsulfonate anion incorporated as part of the phase, which decreases the hydrogen-bond acidity of the column.
One of the most outstanding characteristics observed for the ionic liquid stationary phases was the non-zero value for the b system constant (hydrogen-bond acidity). This inherent acidity contributes toward imparting distinct selectivity to these phases. This is clearly shown by principal component analysis. However, it should be noted that they also present a low inertness to compounds with a high capability to interact through hydrogen-bonding, often observed as poor peak shapes. This makes it difficult to use these columns to quantify low levels of such compounds.
Conclusion
In this work, seven commercial capillary columns that utilize ionic liquid stationary phases were thoroughly studied in order to better understand their retention mechanisms. According to the SPM results, dipole-type interactions (s) and hydrogen-bond basicity interactions (a) were the dominant contributions to retention, hydrogen-bond acidity interactions (b) were moderate, and π–π and n–π interactions (e) were barely significant. It was also revealed they provide a low separation for members of a homologous series, defined as overall dispersive-type (l) interactions.
A 3D PCA plot visually showed that ionic liquid columns fill an empty area of the available selectivity space, which should clearly enhance the separation capacity of the GC technique. It is believed that as a result of the different retention mechanisms involved, the ionic liquid columns studied are highly versatile and have a noteworthy capacity to resolve complex mixtures.

Trademarks
SLB is a registered trademark of Sigma-Aldrich Co. LLC
References
To continue reading please sign in or create an account.
Don't Have An Account?