Aerobic Glycolysis and the Warburg Effect
The Warburg Effect
The Warburg effect is the enhanced conversion of glucose to lactate observed in tumor cells, even in the presence of normal levels of oxygen. Otto Heinrich Warburg demonstrated in 1924 that cancer cells show an increased dependence on glycolysis to meet their energy needs, regardless of whether they were well-oxygenated or not. Converting glucose to lactate, rather than metabolizing it through oxidative phosphorylation in the mitochondria, is far less efficient as less ATP is generated per unit of glucose metabolized. Therefore, a high rate of glucose uptake is required to meet increased energy needs to support rapid tumor progression.
Previously, it was thought the Warburg effect was a consequence of damage to the mitochondria or an adaptation to hypoxic conditions during the early avascular phase of tumor development. Current insight revealed aerobic glycolysis supports various biosynthetic pathways and, consequently, the metabolic requirements for proliferation. The PI3K pathway is considered to be a major determinant of the glycolytic phenotype through AKT1 and mTOR signaling, and subsequent downstream Hypoxy Inducible Factor 1 (HIF-1) transcription factor activation.
Another important pathway is the AMP-activated protein kinase (AMPK) pathway. AMPK is often considered a metabolic checkpoint as it can control cell proliferation when activated under energetic stress, and activation of AMKP results in the inhibition of mTOR activity. Mutations in tumor suppressor genes such as LKB1, involved in AMPK activation, have been identified in certain cancers. Inhibitors of the glycolytic pathway, such as dichloroacetic acid (DCA) and 2-deoxy-d-glucose (2DG) are now being used in clinical studies as potential anticancer agents.
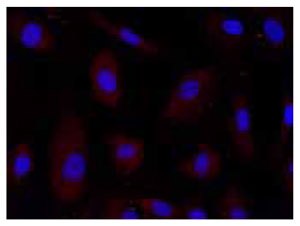
Anti-PGAM2 (38-50): Cat. No. G5672: Immunofluorescence of HUVEC cells using PGAM2 (38-50) (RB), Cat. No. G5672 (red) at a 1:100 dilution, taken at 40× magnification and nuclear staining with Hoescht 33342 (blue). Yale HTCB IF procedure used.
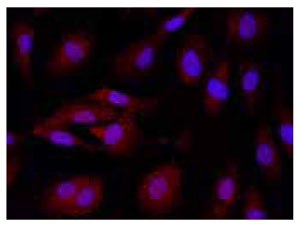
Anti-GPI (211-225): Cat. No. G0422: Immunofluorescence of HUVEC cells using GPI (211-225) (RB), Cat. No. G0422 (red) at a 1:50 dilution, taken at 40× magnification and nuclear staining with Hoescht 33342 (blue). Yale HTCB IF procedure used.
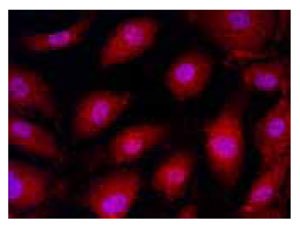
Anti-HK2 (416-429) (RB): Cat. No. H2165: Immunofluorescence of HUVEC cells using HK2 (416-429) (RB), Cat. No. H2165 (red) at a 1:100 dilution, taken at 40× magnification and nuclear staining with Hoescht 33342 (blue). Yale HTCB IF procedure used.
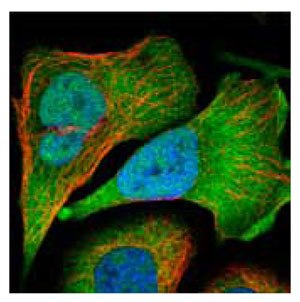
Anti-GPI: Cat. No. HPA024305: Immunofluorescent staining of human cell line U-2 OS shows positivity in nucleus but not nucleoli, plasma membrane, nor cytoplasm.
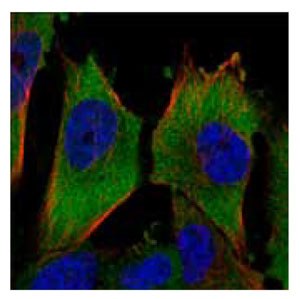
Anti-PKM2: Cat. No. HPA029501: Immunofluorescent staining of human cell line U-251MG shows positivity in cytoplasm.
To continue reading please sign in or create an account.
Don't Have An Account?