Cancer Research Techniques
By focusing on analysis techniques and protocols specific for Cancer Research, we are putting your research needs first. We will be featuring different cancer research tools, providing you with the latest techniques and products necessary for the applications.
Protein Tyrosine Kinase and Phosphatase Expression Profiling in Cancer Research
Tyrosine kinases (PTK) represent only 10% of all protein kinases, although they are the most important protein kinases. PTKs are involved in cell growth signaling. Protein tyrosine phosphatases (PTP) attenuate growth signals generated by the PTKs through catalyzing the tyrosine dephosphorylation step on their substrate proteins. PTPs also are fundamental in cell cycle regulation and activation. Knowledge of the overall expression pattern of PTKs and PTPs in any given cancer cell represents the first step in understanding the sequential events of tumor progression.
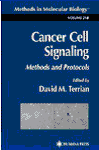
The following functional assay is an excerpt from the book "Cancer Cell Signaling: Methods and Protocols" © 2003 Humana Press. This method is one of several detailed assays featured in this book. The book also contains detailed protocols on the manipulation and detection of signals and protein interactions.
Protein Tyrosine Kinase and Phosphatase Expression Profiling in Human Cancers
Wen-Chang Lin
Institute of Biomedical Sciences, Academia Sinica, Taipei, Taiwan
From Methods in Molecular Biology, Vol. 218, Cancer Cell Signaling: Methods and Protocols pp. 113-125
David M. Terrian, Editor
Humana Press Inc., Totowa NJ, U.S.A.
© 2003 Humana Press
Clarification: The term "we" in this protocol refers to Dr. Lin and his associates.
Materials
1. Total RNA Isolated From Cell Lines or Tissues (25 µg)
2. RT (Reverse Transcription) of First Strand cDNA
- Oligo dT25 anchor primer, 0.5 µg/µL.
- Avian Myeloblastosis Virus Reverse Transcriptase (AMV RT) (R9376), 5 U/µL.
- AMV RT buffer 5X: 250 mM Tris-HCl (T5941), 40 mM MgCl2, 5 mM dithiothreitol (D9779), 150 mM KCl (P9541), pH 8.5.
- dNTP nucleotide mixture (D7295): 10 mM each dATP, dGTP, dTTP, and dCTP.
- RNase inhibitor (ProtectRNA™ RNase Inhibitor 500X concentrate, R2520), 40 U/µL.
- 0.5 M ethylene diamine tetraacetic acid (EDTA) (E7889), pH 8.0.
- CHROMA spin-200 gel filtration column (Alternative: GenElute™ PCR Clean-up Kit, NA1020)
3. PTK/PTP Amplicon Amplification by Polymerase Chain Reaction
- Recombinant Taq DNA polymerase (D4545), 5 U/µL.
- 10X PCR reaction buffer: 200 mM Tris-HCl and 500 mM KCl , pH 8.3.
- 50 mM MgCl2.
- dNTP nucleotide mixture (D7295) 10 mM each dATP, dGTP, dTTP, and dCTP.
- PTK profile degenerate primers.1
Custom DNA oligos
Sense primers:
5’-CAGGTCACCAARRTIDCNGAYTTYGG-3’
5’-CCAGGTCACCAARRTTDCNGAYTTYGG-3’
Antisense primer:
5’-CACAGGTTACCRHAIGMCCAIACRTC-3’ - PTP profile degenerate primers.2
Sense primer:
5’-CAGTGGATCCAARTGYGMIMRRTAYTGGCC-3’
Antisense primer:
5’-CTAGGAATTCCIRYRCCIGCRCTRCARTG-3’ - The mixed bases were defined as follows:
N = A + C + T + G
D = A + T + G
H = A + T + C
V = A + G + C
R = A + G
Y = C + T
M = A + C
and I = deoxyinosine.
4. End-Labeling Sense Primers by T4 Polynucleotide Kinase
- 10X T4 polynucleotide kinase buffer: 700 mM Tris-HCl (T5941), 100 mM MgCl2, 50 mM dithiothreitol (D9779), pH 7.6.
- PTK and PTP sense primers, 10 µM each.
- T4 polynucleotide kinase (P4390), 10 U/µL.
- γ–33P ATP, 10 µCi/µL.
5. PTK/PTP Amplicon Purification, Cloning, and Sequencing (Unlabeled Sense Primer)
- 3% NuSieve® 3:1 agarose gel. (Alternative: Agarose, Wide Range/Standard 3:1, A7431)
- 1X TAE buffer: 40 mM Tris/acetate, 1 mM EDTA , pH 8.0. (TAE buffer, 10X concentrate, T9650)
- 6X gel-loading dye (G7654): 0.25% bromophenol blue, 0.25% xylene cyanol, 15% Polysucrose 400 in H2O.
- DNA fragment extraction kit (GenElute™ Gel Extraction Kit, NA1111).
- TE buffer: 10 mM Tris-HCl, 1 mM EDTA, pH 8.0. (Tris-EDTA buffer, 100X concentrate, T9285)
- T-vector plasmids.
- 10X T4 DNA ligase buffer: 660 mM Tris-HCl (T5941), 50 mM MgCl2, 10 mM dithioerythritol (D8161), 10 mM adenosine triphosphate (ATP) (A2383), pH 7.5.
- T4 DNA ligase (D2886), 1 U/μL.
- Competent bacterial cells, high efficiency (GC5 Competent cells or GC10 Competent cells, G2794).
- Plasmid DNA extraction kit (GenElute™ Plasmid Miniprep Kit, PLN70).
- GenBank, BLAST: http://www.ncbi.nlm.nih.gov/.
6. PTK/PTP Amplicon Purification, Restriction Enzyme Digestion, and Gel Display (Labeled Sense Primer)
- 3% NuSieve® 3:1 agarose gel. (Alternative: Agarose, Wide Range/Standard 3:1, A7431)
- 1X TAE buffer: 40 mM Tris/acetate, 1 mM EDTA, pH 8.0. (TAE buffer, 10X concentrate, T9650)
- 6X gel-loading dye (G7654): 0.25% bromophenol blue, 0.25% xylene cyanol, 15% Polysucrose 400 in H2O.
- DNA fragment extraction kit (GenElute™ Gel Extraction Kit, NA1111).
- TE buffer: 10 mM Tris-HCl, 1 mM EDTA, pH 8.0. (Tris-EDTA buffer, 100X concentrate, T9285)
- 10X restriction enzyme buffer.
- Restriction enzyme panel: Alu I (R6885), Cfo I (R1761), Dde I (R4256), Hae III (R5628), Hinf I, Mnl I, Msp I (R4506), Sau3al, Sau96I, Scrf I (R7888), Tru9I, and Rsa I (R4756).
- Formamide loading dye: 100% formamide (F7508), 0.025% bromophenol blue (B0126), 0.025% xylene cyanol (X4126).
- 7% polyacrylamide/urea sequencing gel.
- 35S-dATP labeled pre-determined DNA sequence ladder as size marker.
Methods
1. RT of First Strand cDNA
- 25 µg total RNA is precipitated, centrifuged and dissolved in 10 µL DEPC H2O.
- 1 µL oligo dT primer and 5 µL DEPC H2O are added to the RNA tube, and incubated at 65 °C in a water bath for 10 min. Transfer the reaction tube onto ice following the 65 °C RNA denaturation step.
- Add 5 µL 5X AMV RT buffer, 2.5 µL dNTP nucleotide mixture, 0.5 µL RNase inhibitor, and 1 µL AMV RT enzyme to bring up to a total 25 µL reaction volume. Mix well and spin briefly in an Eppendorf centrifuge.
- Incubate at 42 °C in a water bath for 30 min. Stop the reaction by adding 1 µL 0.5 M EDTA solution.
- Bring the total volume to 65 µL by adding DEPC H2O, and remove RT enzymes by phenol/chloroform (P1944) extraction once and chloroform (C7559) extraction once. Transfer the aqueous phase to a fresh Eppendorf tube.
- Spin the CHROMA spin-200 column to remove buffer, and load the first strand RT reaction mixture onto the CHROMA spin-200 column. Centrifuge the column again to separate the first strand cDNA products and unincorporated dNTP mixture and primers. Collect the effluent in a fresh Eppendorf tube, and store the first strand RT cDNA template at –80 °C if necessary.
2. PTK/PTP Amplicon Amplification by PCR
- Add the following components to a sterile 0.2 mL thin-wall PCR tube sitting on ice:
| 5.0 µL 0.8 µL 1.0 µL 5.0 µL 5.0 µL 5.0 µL |
- Mix well and overlay with 50 µL mineral oil. Centrifuge briefly to collect the content to the bottom.
- Incubate the reaction tube in a PCR thermal cycler at 96 °C for 3 min. to completely denature the template.
- Set the thermal cycler at 68 °C following the denaturation step.
- Add 1 µL Taq DNA polymerase to the bottom of reaction mixture, and mix gently.
- Perform four cycles of PCR amplification as follows:
| 93 °C for 45 s 44 °C for 90 s 72 °C for 12 s |
- Perform an additional 26 cycles of PCR amplification as follows:
| 93 °C for 45 s 56 °C for 90 s 72 °C for 15 s |
- Incubate for an additional 10 min at 72 °C and maintain the reaction at 4 °C until subsequent purification.
3. End-Labeling Sense Primers by T4 Polynucleotide Kinase
- Mix 2 µL sense primer, 1 µL 10X T4 polynucleotide kinase buffer, 5 µL γ-33P ATP, 0.5 µL T4 polynucleotide kinase, and 1.5 µL H2O into a sterilized Eppendorf tube.
- Incubate the reaction mixture at 37 °C in a water bath for 30 min., and then incubate at 65 °C in a water bath for another 5 min. to inactivate the T4 polynucleotide kinase enzyme activity.
- The labeled sense primers were used as 10X stock solution for the PCR experiments.
4. PTK/PTP Amplicon Purification, Cloning, and Sequencing (Unlabeled Sense Primer)
- Prepare 3% NuSieve® 3:1 agarose gel in 1X TAE buffer.
- Load the amplified PCR products into agarose gel by mixing with loading dye.
- After running the gel, stain with 1 µg/mL ethidium bromide (E7637), and excise the 170 bp amplicon DNA fragments from gel.
- Purify DNA fragments from gel following the instructions of DNA fragment extraction kits (GenElute™ Gel Extraction Kit, NA1111).
- Repeat the extraction steps to remove possible containment. Amplified PTK/PTP amplicon fragments are then precipitated with ethanol (E7023), centrifuged, and dissolved in TE buffer.
- Mix 1 µL PTK/PTP amplicon DNA fragments with 1 µL 10X T4 DNA ligase buffer, 2 µL T-vector plasmid, 1 µL T4 DNA ligase and 6 µL H2O. Incubate the ligation mixture overnight at 15 °C.
- Perform transformation procedure with suitable competent Escherichia coli cells, select antibiotic resistant bacterial colonies and isolate plasmid DNA from randomly selected colonies.
- PTK/PTP insert sequences of each plasmid are determined by DNA autosequencer. The sequences obtained can be compared with GenBank database sequences from National Center for Biotechnology Information using the BLAST program to identify each PTK/PTP gene expressed.
- PTK/PTP expression profiles can be established by the number of different kinase/ phosphatase clones identified.
5. PTK/PTP Amplicon Purification, Restriction Enzyme Digestion, and Gel Display (Labeled Sense Primer, Note 2)
- Prepare 3% NuSieve® 3:1 agarose gel in 1X TAE buffer.
- Load the amplified PCR products into agarose gel by mixing with loading dye.
- After running the gel, stain with 1 µg/mL ethidium bromide (E7637), and excise the 170 bp amplicon DNA fragments from gel.
- Purify DNA fragments from gel following the instructions of DNA fragment extraction kits (GenElute™ Gel Extraction Kit, NA1111).
- Repeat the extraction steps to remove possible containments. Amplified PTK/PTP amplicon fragments are then precipitated with ethanol (E7023), centrifuged, and dissolved in TE buffer.
- Determine the radioactivity of recovered PTK/PTP DNA fragments, and adjust the final volume to 104 cpm/µL.
- Equal amounts (at least 40,000 cpm) of the eluted PTK/PTP amplicon are then digested 60 min. with respective restriction enzymes listed in the panel (Materials 6, Item 7) under optimal restriction enzyme conditions.
- 6.6 µL formamide loading dye is added to the digested products and incubated at 65 °C for 7 min. before loading onto gel.
- 4 µL of final product is applied to each lane in 0.7% denaturing sequencing polyacrylamide gel. A sequencing reaction product (35S-label, and single-track sequencing only) with a predetermined sequence template is used as a standard for fragment size throughout the profile analysis. Any known sequence will be useful, because we use it as a size standard. (Notes 3-5)
- Following electrophoresis, the gel is dried and exposed to a X-ray film or processed by a phosphoimager.
- The amplified PTK products are digested with Mwo I restriction enzyme and separated on a sequencing gel. Samples from multiple tissues could be displayed on a single sequencing gel; thus, we could effectively screen all known human PTKs/PTPs in a short period of time.
- From the pre-established bioinformatics database of human PTKs/PTPs digested with different restriction enzymes, individual tyrosine kinases were identified based on their respective characteristic restriction fragment sizes on the exposed X-ray films or in the phosphoimager-processed files.
- PTK/PTP expression profiles can be established by the radiation dose unit numbers of different kinase/phosphatase identified. (Note 6)
Notes
- The catalytic domains of PTK and PTP can be divided into a number of smaller sub-domains that represent localized regions of high conservation.3,4 By using degenerate primers from these regions, as well as the RT-PCR strategy, we could easily amplify most PTK/PTP genes expressed in cells, if not all PTKs/PTPs. The first PTK/PTP profiling method (Methods 2) required cloning and sequencing hundreds of clones following the RT-PCR reactions.5, 6 Although it was an effective approach, it was time consuming for sequencing hundreds of clones. In addition, this protocol could not generate a more comprehensive and quantitative PTK/PTP profile until thousands of clones were sequenced. However, it is suitable for screening novel PTK/PTP genes in organisms without comprehensive genome sequence information as previously demonstrated by others.7,8,9
- In this second PTK/PTP display approach (Methods 5), the expression level of PTK kinase genes could be represented by the intensity of each recognized band. This protocol is established on the bioinformatic restriction digest databases, which require the sequences of almost all PTKs/PTPs. Otherwise, there will be many unknown fragments on the gel and thus severely diminishes the effectiveness of this display approach. Although one can excise the fragments of interest from gels and identify them by cloning methods,10 it is time consuming and less effective. Because it did not employ the previously used cloning and screening procedures, this display method can be quantitative and more representative by utilizing the radiolabeled primers.1,11
- Because the radioactive label is only on the forward primers, each radioactive signal is generated from one labeled primer. Another advantage of this method is that one can perform multiple PTK profiles on several cell lines or tissues at the same time, whereas it would be difficult to perform large amounts of screening with the previous cloning and sequencing method. As discussed before, a comprehensive database is essential for this display approach, which might be useful for human or mouse PTK/PTP profiling. One has to screen the genome sequence or EST databases to generate the restriction digest databases.12 We have now implemented bioinformatic programs to automatically screen the genome and generate restriction digest databases for display purposes. Another issue that could be resolved by bioinformatic tools is single nucleotide polymorphism (SNP) within the PTKs/PTPs.
- Because the display approach depends on the restriction enzyme recognition, SNPs located in the amplified PTK/PTP regions will affect the restriction enzyme recognition and generate different digestion fragments. This is similar to restriction fragment length polymorphism. Generally, it is not an important issue for the PTK/PTP display approach, because we are using a panel of restriction enzymes to determine the identity of each PTK/PTP gene, not just one enzyme. In addition, similar size restriction fragments could represent several different kinases, and alternative restriction enzymes would be necessary to identify single gene specific restriction fragments. It was calculated that about 15-20 restriction enzymes (4-base or 5-base hitters) were required to cover all genes here. With the aid of bioinformatic software, we might be able to include SNP information in the databases in order to generate a better PTK/PTP profile. With completion of the human genome project, we could examine the entire human transcriptome and learn more about the composition and evolution of gene families in the human genome. This will greatly assist this display approach, because all restriction fragments or patterns could be assigned to every known gene.
- Additional improvements can be achieved in primer design. We use the degenerate primers based on the conservative motifs of PTKs/PTPs to cover all known and unknown genes, and specificity is compromised with the degenerate primer design. Because we now have all the human genome sequences, new human profile primers can be designed to increase specificity and reduce cross-hybridization with other serine/threonine kinases or phosphatases.
- One major drawback for this display protocol is the short half-life of radioactive isotope labeled primers. In the future, with the addition of fluorescent-labeled PCR primers, we can also include internal MW markers, control genes tagged with different color dyes in the same reaction. This will extend the usage of labeled primers, because there is no isotope half-life and re-labeling considerations. Quantitation of each band was easier and less time consuming with fluorescent-labeled primers. With 33P labels, exposure time to film or phosphoplate ranged from three to seven days. 32P can be used to reduce the process time,10 however, gel resolution is much reduced owing to the strong 32P radioactivity. Analysis could be performed immediately following gel separation with fluorescent labels on autosequencers.
References
To continue reading please sign in or create an account.
Don't Have An Account?