HS-SPME/GC-MS Method Development for the Qualitative Analysis of Volatile Compoundsin Fresh Perilla Leaves Using Different SPME Fibers
Deyny Mendivelso-Pérez, Robert E. Shirey, Olga Shimelis, R&D Merck
Abstract
Solid phase microextraction (SPME) fibers were used in a high-throughput headspace-solid phase microextraction gas chromatography-mass spectrometry (HS-SPME/GC-MS) method for analysis of volatile compounds present in perilla herbs acquired from local markets. The main components found in the sample were perilla ketone, (-)-trans-caryophyllene, a-humulene, and (Z, E)-α-farnesene independently of the type of coating tested. DVB/PDMS coating on Nitinol core showed the best performance with regards to the number and amount of extracted compounds and the lack of carry-over effects.
Section Overview
Introduction
Perilla (Perilla Frutescens) is an annual herb that belongs to the Lamiaceae family with a characteristic aroma and flavor. The leaves are usually used as a flavoring ingredient in cooking and traditional herbal medicine. 1-3 Volatile composition of perilla leaves varies across species, geographical origins, and growing environment conditions. Perilla aldehyde and perilla ketones (perilla ketone, egoma ketone and isoegoma ketone) are characteristic aroma-active compounds in the leaves.2,4-7 Although perilla aldehyde is used as a food additive, studies have demonstrated that perilla ketones are potent pulmonary agents for laboratory animals and livestock. 5,7 Given that the toxic dose of perilla ketone to humans is unknown, perilla species with high content of perilla ketone are considered not suitable for human consumption.4,5 Perilla ketone-containing species are of high concern to farmers whose grazing animals are prone to ingest the perilla plant that usually grows as a weed in pastures. Specifically, perilla plant is a common weed found in southeastern states of the United States. In these states, the number of reported poisoned and dead cattle increase during the late summer and early fall, when animals are forced to graze this plant when forage is scarcer. Moreover, hay contaminated with dried perilla represents a high risk for livestock.8 A rigorous screening of the volatile profile of perilla leaves is required for presence of perilla ketone given its demonstrated harmful effect in animals and potential hazard to the human health.

Figure 1.Main aroma-active compounds present in Perilla Frutescens species.
Experimental
Fresh Perilla samples used in this study were purchased from two local grocery stores and kept under refrigeration until further analysis. During sample preparation, leaves were placed on dry ice, and then the frozen leaves were homogenized using a food processor until a finely ground sample was obtained as illustrated in Figure 2. The homogenized sample was stored under CO2 until extraction. Approximately 0.05 g of homogenized sample was transferred into 10 mL screw cap vials and stored on dry ice until HS-SPME/GC-MS analysis. SPME fibers of four different coating chemistries were employed to obtain a wide volatiles profile and to select the optimal fiber for extraction of perilla ketone and perilla aldehyde. Additionally, other volatile components of dried perilla leaves were also identified and analyzed. For this experiment, perilla leaves were dried at room temperature until constant weight was observed. Approximately 0.05 g of the dried samples were transferred to a 10 mL headspace vial.
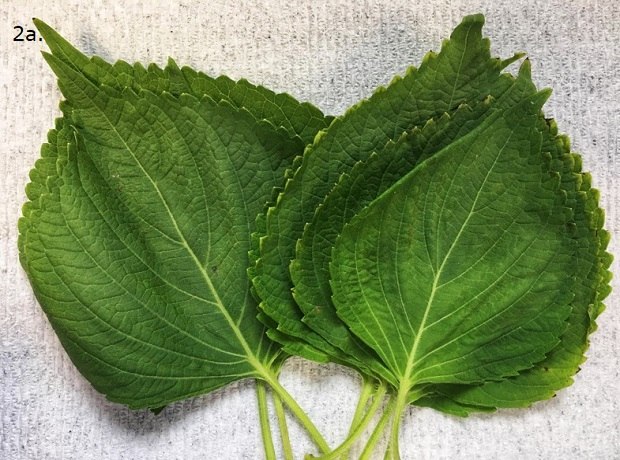
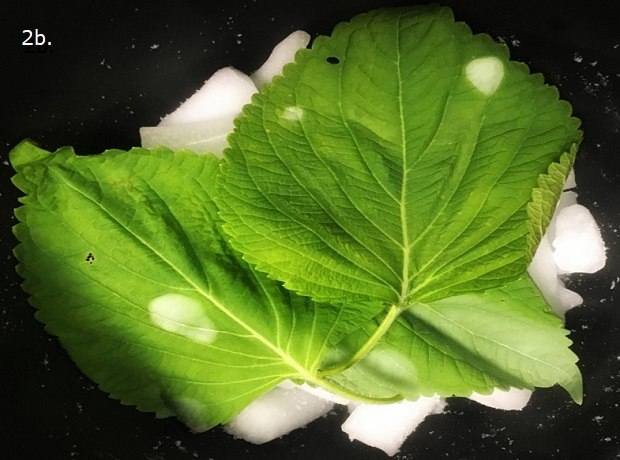
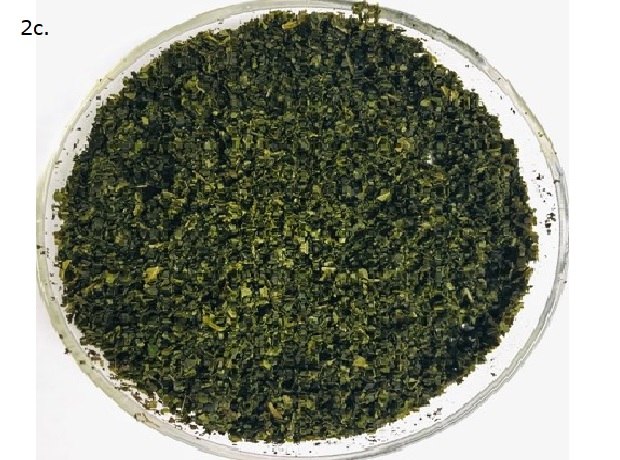
Figure 2. Sample preparation process. (1) Fresh Perilla Leaves; (2) Sample freezing over CO2 pellets; (3) Sample after homogenization process.
The parameters for HS-SPME and GC-MS optimized methods are listed in Tables 1 and 2. Peak identifications were assigned using MS spectral matching against reference spectra in the Wiley and NIST libraries. Additionally, confirmatory identification was done by comparing the MS spectra of the sample with analytical compound standards.
Sample/matrix: | 0.05 g of fresh homogenized Perilla leaves in a 10 mL headspace vial 0.05 g of dried perilla leaves in a 10 mL headspace vial |
---|---|
Autosampler: | Gerstel MPS II with cooled tray holder |
SPME Fibers: | PDMS on Fused silica core, 100 µm, 23 g, 1 cm DVB/PDMS on Nitinol core, 65 µm, 23 g, 1 cm CAR/PDMS on Nitinol Core, 75 µm, 23 g, 1 cm DVB/CAR/PDMS on StableFlex core, 50/30 µm, 23 g, 1 cm |
Incubation: | 30°C for 2 min with agitation |
Agitation: | Rotation speed of sample at 250 rpm |
Extraction: | Headspace for 10 min at 30 oC with agitation |
Desorption: | 3 min at 300°C for CAR/PDMS Fiber 3 min at 270 °C for DVB/CAR/PDMS, PDMS, and DVB-PDMS Fibers |
Fiber post-baked: | 2 min at 300°C for CAR/PDMS Fiber 2 min at 270 °C for DVB/CAR/PDMS Fiber 2 min at 250 °C for PDMS and DVB/PDMS Fibers |
Column: | SLB®-5ms 20 m x 0.18 mm, df=0.18 µm (28564-U) |
---|---|
Oven: | 40°C for 2 minutes, then 10°C/min to 120°C, then 20°C/min to reach 280°C. Hold at 280°C for 0.2 minutes |
Carrier gas: | Helium, 1 mL/min constant flow |
Detector: | MS Quadrupole, full scan, m/z = 45-400, Source at 230°C, Quad at 150°C, Electron energy at 70 eV |
MSD Interface: | 325°C |
Inlet: | 300°C for CAR/PDMS Fiber 270 °C for DVB/CAR/PDMS, PDMS, and DVB/PDMS Fibers |
Liner: | 0.75 mm ID SPME |
Injector: | Split (10:1) |
Standard solutions: | Limonene, Linalool, Perilla ketone, Perilla aldehyde, (-)-trans-caryophyllene, α-Humulene (all 50 ppb in methanol) |
Results and Discussion
The volatile profile of fresh perilla leaves obtained from the four different SPME coatings shows the presence of 1-octen-2-ol, 3-octanol, perilla ketone, egoma ketone, isoegoma ketone, (-)-trans-caryophyllene, α-humulene, and (Z, E)-α-farnesene, as shown in Figure 3. It can be observed that DVB/PDMS and PDMS fibers extracted a wider range of volatile components. However, DVB/PDMS fiber coating was much more effective in the extraction and desorption of the volatile components compared to the other fiber chemistries. Carboxen-containing fibers presented a carryover effect due to a higher retention of perilla ketone.
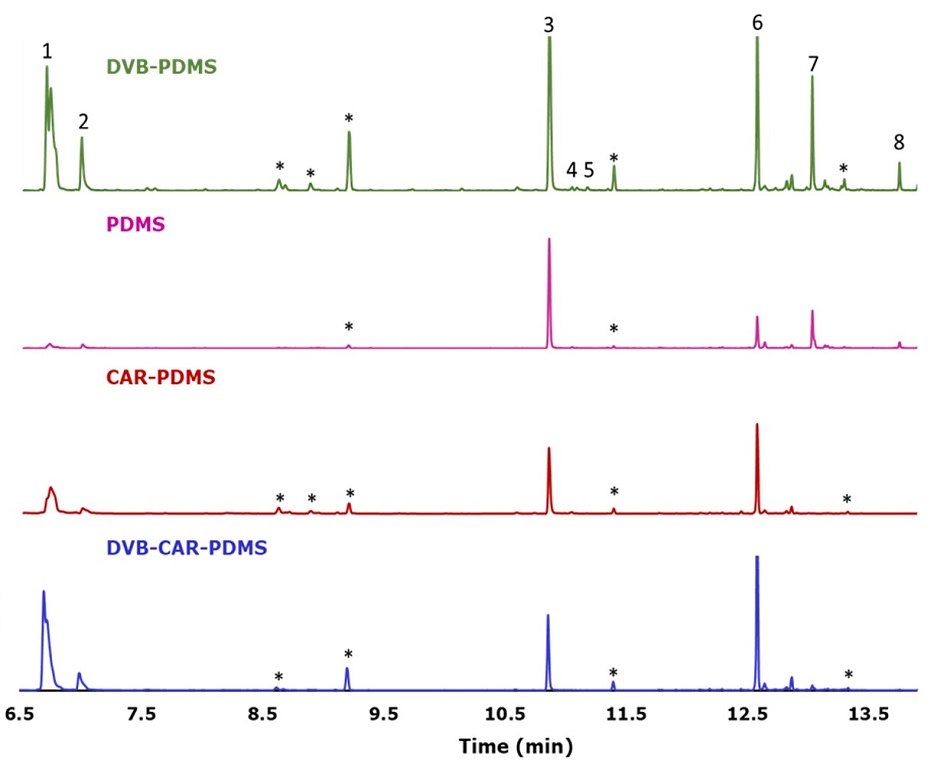
Figure 3.GC-MS chromatograms of fresh Perilla leaves obtained different SPME coatings. (1) 1-Octen-2-ol; (2) 3-octanol; (3) perilla ketone; (4) egoma ketone; (5) isoegoma ketone; (6) (-)-trans-caryophyllene; (7) α-humulene; (8) (Z,E)-α-Farnesene; (*) fiber peak. All chromatograms were adjusted to the same intensity.
HS-SPME-GC-MS relative content results in Figure 4 suggest that the tested samples has relatively high content (approx. 10-50%) of perilla ketone. Interestingly, egoma ketone and isoegoma ketone were present in low concentrations (<1%).
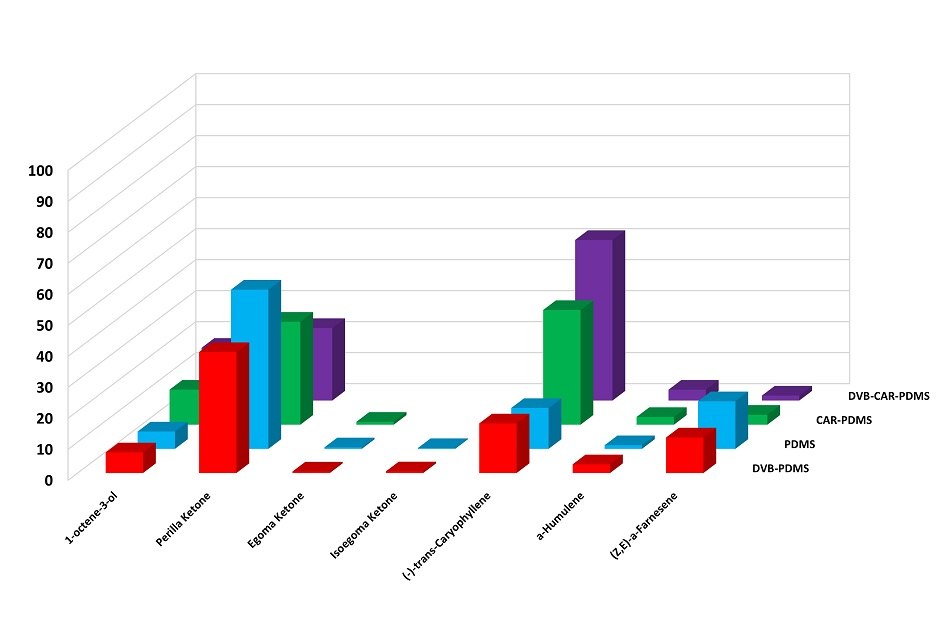
Figure 4.Comparison of the main components present in fresh leaves obtained by HS-SPME. 100% was the total of all peaks in the chromatogram except the background fiber peaks.
The volatile profile of dried perilla leaves was obtained using a DVB/PDMS fiber coating on a nitinol core. The compounds that were found both in fresh and dried leaves are shown in Figure 5. These results suggest that the drying process effected the relative content of the main volatile components, where the volatile profile is composed of 1-octen-3-ol (21%), perilla ketone (56%), (-)-trans-caryophyllene (1%), and α-humulene (0.9 %). In addition, other minor components were identified in the volatile profile of dried perilla leaves not seen in the fresh leave, e.g., benzaldehyde, phenylethyl alcohol, eugenol. Perilla ketone governs the volatile composition in dried samples. Figure 6 shows a chromatogram of the analytical standard used for confirmation of the identity of main volatile compounds present in perilla leaves and extracted with a DVB/PDMS fiber.
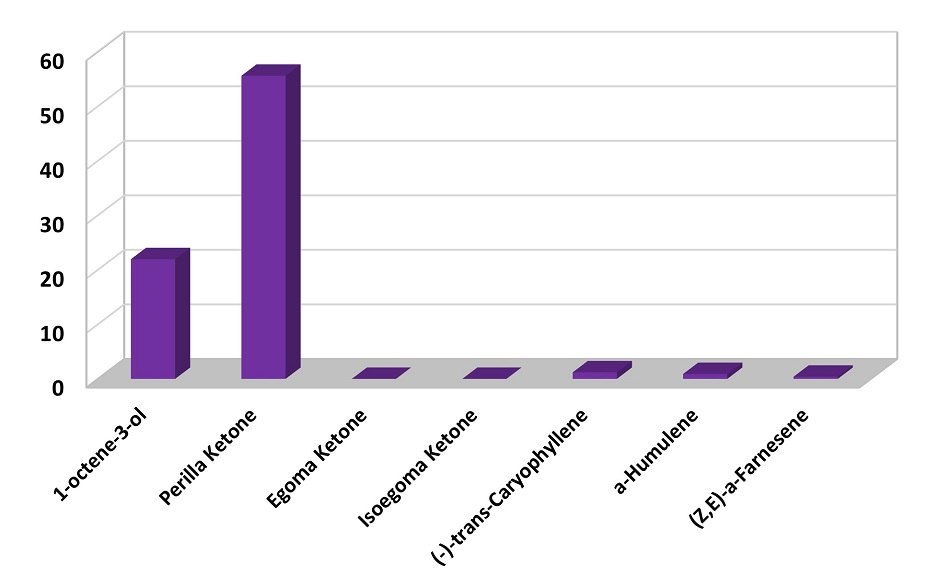
Figure 5.Main volatile components present in dried perilla leaves. 100% was the total of all peak areas in the chromatogram except the background fiber peaks.
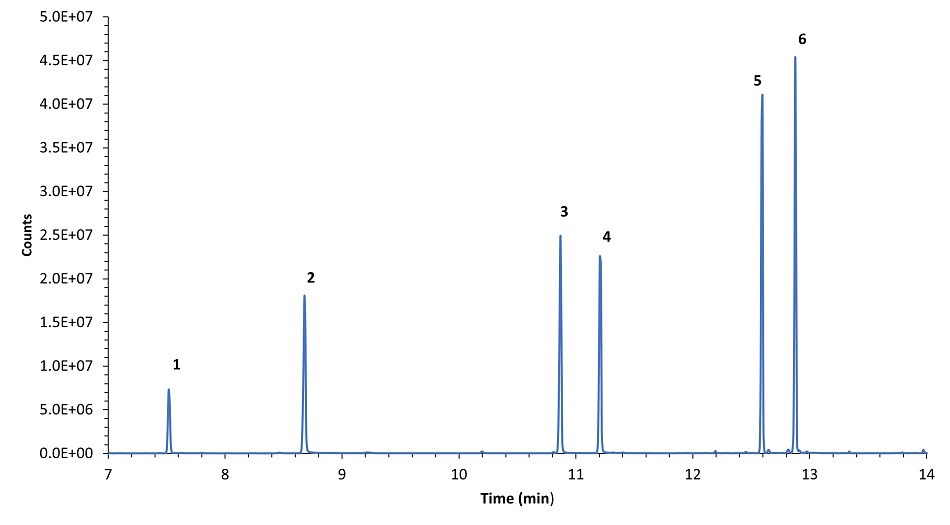
Figure 6.Chromatograms of analytical standards obtained under the sampling conditions using 50 ppb standard in methanol. SPME coating: DVB/PDMS. 1. Limonene, 2. Linalool, 3. Perilla Ketone, 4. Perilla Aldehyde, 5. (-)-trans-Caryophyllene, 6. α-Humulene
Conclusion
This application has proposed a HS-SPME/GC-MS high-throughput method to obtain the volatile profile in Perilla leaves using a low extraction temperature. Additionally, it has been demonstrated that divinylbenzene-polydimethylsiloxane (DVB/PDMS) SPME fiber on the Nitinol core is the best choice for the extraction of volatile compounds due to its effectiveness in both extraction and desorption of the volatile components compared to polydimethylsiloxane (PDMS), Carboxen®-polydimethylsiloxane (CAR/PDMS), and divinylbenzene-Carboxen®-polydimethylsiloxane (DVB/CAR/PDMS), with absent of carryover effect. Furthermore, the effect of drying process on the volatile profile in dried perilla was characterized. The results for the samples tested showed the presence of perilla ketone in both fresh and dried perilla leaves.
To continue reading please sign in or create an account.
Don't Have An Account?