Cost Modeling Vaccine Manufacturing: Estimate Production Costs for mRNA and other Vaccine Modalities
Joséphine Cheng, Senior Consultant, Traditional Modalities Asia, Bioprocessing Strategy, Jérome Dalin, Senior Consultant, BioProcessing Strategy Development, Biopharma 4.0, Cory Peterson, Strategy Analytics and Intelligence, Valentin Delor, Senior Strategy & Market Intelligence Analyst
There is a wide range of vaccine modalities that can be leveraged against infectious pathogens, each having its own set of advantages, disadvantages, and production costs. To determine which is best for a particular application, it is essential to understand, evaluate, and optimize costs to maximize production efficiency. This article describes the use of a custom-designed cost model to estimate the economics of vaccine manufacturing when using different modalities.
- Use of Cost Modeling to Understand Processes
- Components of the Vaccine Production Cost Model
- Analysis and Comparison of Cost Structures, Cost Per Dose, and Operational Effectiveness
- Qualitative and Quantitative Comparison of Vaccine Modalities
- Cost Modeling Provides Critical Decision-Making Insights
- Explore Related Products
- Discover Related Applications
Use of Cost Modeling to Understand Processes, Simulate Bottlenecks, and Optimize Production Efficiency
Cost modeling is a powerful tool that be leveraged to better understand vaccine manufacturing processes, simulate bottlenecks, and optimize production efficiency. The cost model described below considers direct and indirect costs across the vaccine manufacturing process, from research to GMP manufacturing. Other costs such as R&D, marketing and licensing, packaging, and distribution, would be separate from the modeling calculation, as these costs can vary significantly depending on each product.
With such detailed modeling, it is possible to determine the cost to manufacture a vaccine using different modalities and how decisions related to the process, such as scale and specific technologies, can impact these costs. Cost modeling can also provide valuable guidance related to facility design for engineering teams.
Cost modeling can be applied throughout the vaccine manufacturing process to understand and identify the biggest cost contributors to the process. Sensitivity and what-if analyses can be performed to determine the cost impact of changes to demand and titer, and whether bottlenecks will be introduced by these changes. Models can also be used to run scenario simulations and evaluate proposed process changes on overall costs, quantify tradeoffs, quantify how costs shift with increased scale, and whether there may be limitations in equipment. Simulating process scheduling to optimize facility and labor scheduling is also possible.
There are limitations to cost modeling, however. It can’t predict if the given approach will work from a technical standpoint and does not use absolute costs with high accuracy. Cost models cannot address elements of uncertainty including market changes, acknowledged and mystery unknowns, and the potential impact of technical innovations. As market dynamics and technology evolve, cost models require regular calibration to remain relevant.
Components of the Vaccine Production Cost Model
The cost model described below encompasses capital, labor, materials, consumables, and other costs, which are five key parameters of manufacturing for a variety of vaccine modalities (Figure 1). Manufacturing costs, which reside between the lengthy and highly variable costs of process development and commercialization, are more easily defined and therefore more amenable to being assessed via cost modeling.
The model was developed using BioSolve software (Biopharm Services) and was based on inputs related to the process flow and parameters, cost of materials, and other assumptions. Model inputs were based on published information; mass/material balances, sizing for consumables, and equipment were based on anticipated process volumes, along with time, labor, floorspace, and utilities, to calculate the cost of manufacturing, capital expenses, and the relevant bills of material.
Other Costs
- Insurance
- Engineering and spare parts
- Utlities
Labor
- Production staff
- Quality staff
- Other staff
Consumables
- Filters, membranes
- Chromatography resins
- Single-use components
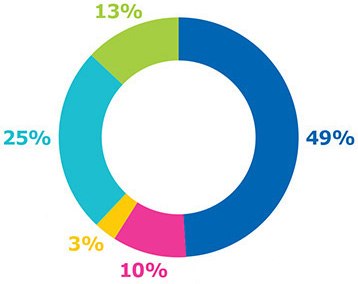
Figure 1.Manufacturing-related parameters included in the cost model.
Capital
- Equipment cost
- Capital Estimate (installation, pipework, HVAC, power, building)
- Validation
Materials
- Media, buffer
- Cleaning chemicals (solid, PW/WFI)
Analysis and Comparison of Cost Structures, Cost per Dose, and Operational Effectiveness of Different Vaccine Modalities
The objective of this initiative was to analyze and compare the cost structures and operational effectiveness of producing a range of vaccine modalities from the more traditional to those on the leading edge of innovation. The model was also used to reveal steps that might be possible bottlenecks based on the contribution to overall costs, and to explore the impact of legacy processes and single-use technologies on the cost structure.
Results: Cost per Dose Comparison of Single-Use and Legacy Manufacturing Technology
Figure 2 provides a cost comparison of stainless steel and single-use processes. Cost per dose was generally higher using stainless steel equipment, mainly due to the much higher cost contributed by capital investment and labor, which overshadowed the savings on consumables and materials. Labor costs included a range of activities such as steam-in-place and cleaning-in-place procedures, buffer preparation, and validations. While a lower utilization rate increased the impact of capital cost, simulation of scenarios can be used to identify an optimized alignment of cost savings and production efficiency.
The cost distribution for a legacy process and single-use process under low and high facility utilization rates is shown in Figure 2 for inactivated and protein subunit viruses, two common modalities used in marketed products.
Cost distribution among a legacy process and a single-use process were compared under low and high facility utilization rates. As shown in Figures 2A and 2B, the modern single-use process had a lower overall cost per dose, due to a reduction in labor and capital and a higher yield in modern single-use processes. As a result, the number of batches was lower for the single-use process which translated to lower consumables cost. A comparison of the modern protein subunit processes in Figures 2C and 2D demonstrated that the yield was the same for both legacy and single-use processes. As the facility utilization rate increased to 80-90%, the cost per dose was comparable; there was a reduction in capital and labor cost for the single use process and a higher percentage of consumables costs (2D).
The single-use process has a lower overall cost per dose, due to a reduction in labor and capital and a higher yield from this more modern process for production of inactivated virus vaccines (Figures 2A and B). This results in a smaller number of batches and thus the lower consumable cost. For protein subunits, the yield is the same for both legacy and single-use processes (Figures 2C and D). As the facility utilization rate increased to 80-90%, the cost difference was reduced and nearly comparable. The cost for consumables, however, was higher while capital and labor were lower in the single-use processes.
Predicting demand for a vaccine can be difficult, leading to changes in facility output and utilization. Single-use technology offers a more flexible approach for meeting demand and reducing the initial capital spend, while maintaining a low cost of goods. Subsequent data sets focus on single-use processes and an mRNA platform; a 10M annual dose production volume was used to compare costs across different vaccine modalities.
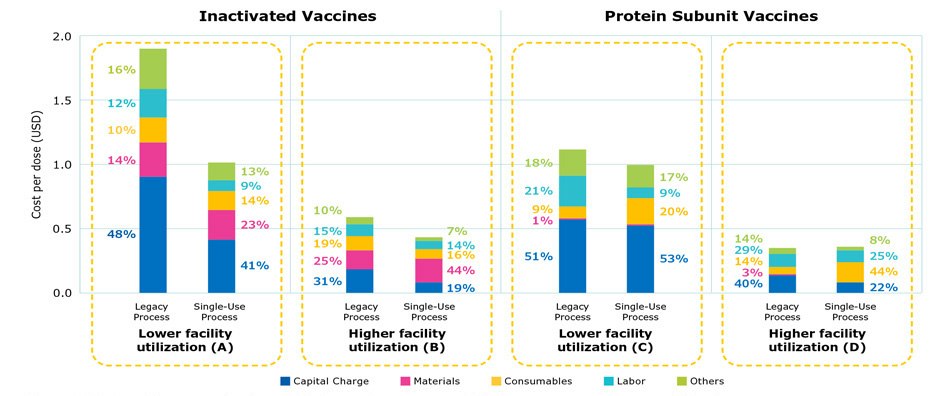
Figure 2.Cost per dose comparing legacy stainless steel processes and single-use processes at commercial scale.
Results: Differences in Cost of Goods and Cost per Dose based on Vaccine Modality
Figure 3 shows the cost per dose by modality at commercial scale production with fully single-use processes. For all modalities, labor costs averaged close to 10% and the contribution of consumables, such as chromatography resin or single-use materials, increased as the number of batches increased. As shown by the results, overall cost per dose was highest for mRNA vaccines, followed by traditional inactivated vaccines, protein subunit vaccines, and virus-like-particle vaccines.
Inactivated vaccines have similar costs related to materials and consumables; capital expenses represent about one-third of the total cost. Virus-like particle (VLP) vaccines produced using insect cell systems have lower consumable costs due to higher titers and fewer batches being needed to achieve the same annual dose volume requirement compared to CHO cell-based protein subunit platforms. mRNA vaccines had the lowest capital and labor costs, due to having the smallest production footprint and a simpler processing workflow.
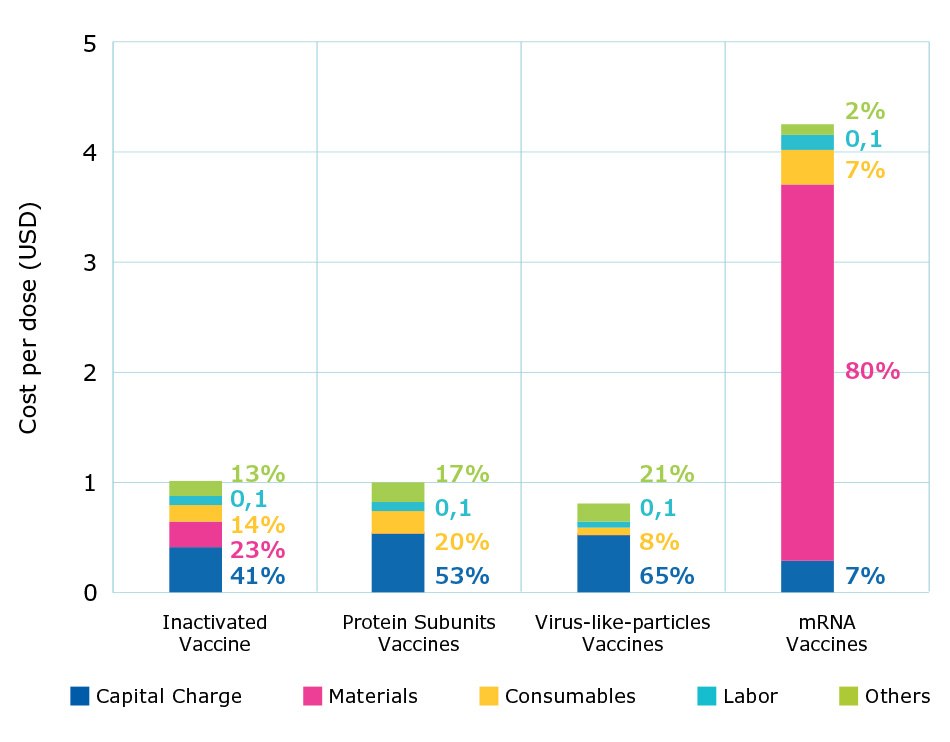
Figure 3.Percent contribution of individual costs and cost per dose by modality at commercial scales.
Results: Contribution of Unit Operations to Overall Cost of Different Vaccine Modalities
The model was next used to identify the cost of each unit operation for different vaccine modalities. Figure 4 compares the cost structure of inactivated and mRNA vaccines as an example. When considering overall manufacturing cost distribution, the capital charge for inactivated vaccines is approximately 41%, compared to 7% for mRNA vaccines; material cost is approximately 23% for inactivated vaccines and 81% for mRNA vaccines. The difference in cost distribution reflects the fact that initial capital expenses, the scale of hardware systems, and facility footprint are higher with inactivated vaccines, compared with mRNA vaccines. With mRNA vaccines, most of the cost results from materials required for day-to-day operation.
The costliest operations in the mRNA process are plasmid linearization, transcription, and enzymatic reaction steps, while other costs are relatively minimal. With inactivated vaccines, use of endonuclease to digest host cell DNA is costly and increases the overall cost. Understanding the impact of various unit operations helps direct attention to the areas of the workflow which can be further optimized to reduce costs.
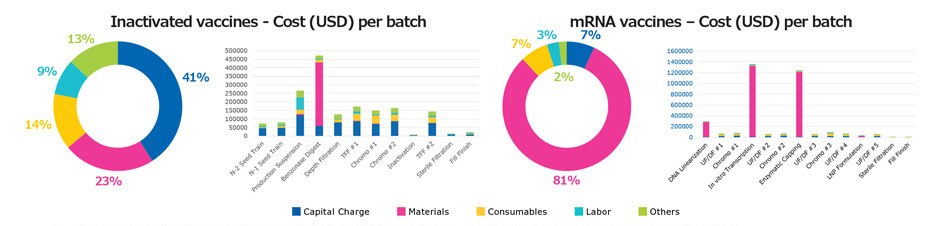
Figure 4.Comparison of cost distribution among major unit operations for inactivated and mRNA vaccine modalities.
Results: Contribution of Materials and Technologies to Overall Cost of Different Vaccine Modalities
Figure 5 shows the contribution of different materials and technologies including cell culture media, biopharma material, clarification, chromatography, ultrafiltration, virus filtration, aseptic filtration, and single use bags and tubing; capital expenses and labor were not included. This analysis provides insight into which technology offers the best opportunity for optimization and cost reduction.
Single-use consumables were the largest cost contributors, for example, ranging from 45% to 90% depending on the modality. Costs of processing materials, such as endonuclease enzymes, were the biggest contributors for virus-based vaccines for day-to-day operations.
For protein subunit vaccines and VLPs, the cost for chromatography and clarification filters stands out. For mRNA vaccines, biopharma materials represent more than 90% of operating costs. To reduce costs for producing mRNA, less expensive enzymes or nucleotides could be used. Alternatively, the mRNA vaccine dose could be reduced using self-amplifying mRNA; with a lower overall dose volume, cost per dose can be reduced.
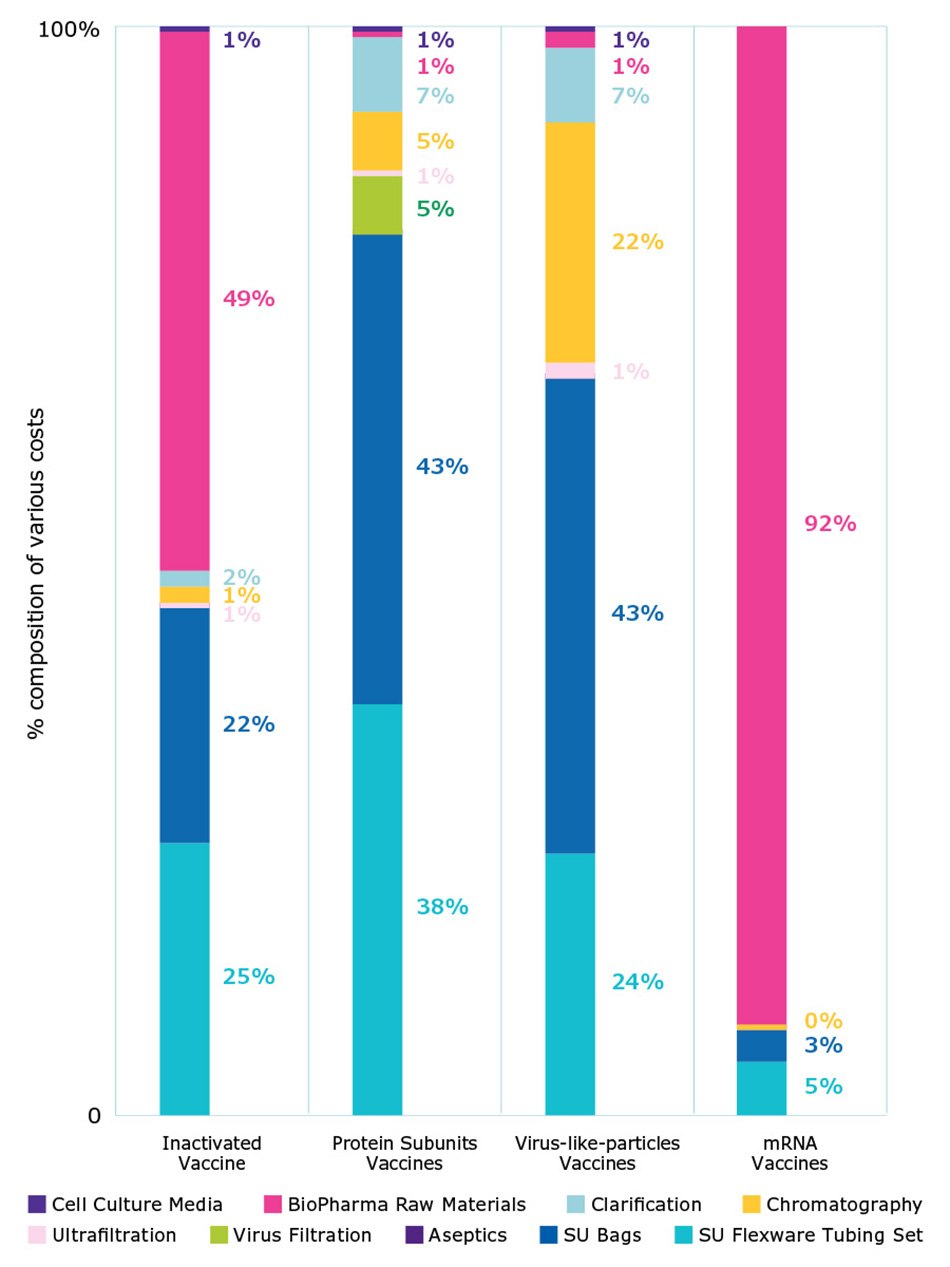
Figure 5.Comparison of cost of goods for materials and consumables in routine manufacturing.
Qualitative and Quantitative Comparison of Vaccine Modalities
In addition to cost modeling, several qualitative and quantitative aspects related to the production of different vaccine modalities should be considered when choosing a development path (Table 1).
For example, inactivated vaccines have an excellent regulatory record, good product stability, process development is relatively straightforward, and the immunogenicity mechanism is well understood. These vaccines can, however, pose a risk for reverse virulence, creating a greater biosafety hazard for operators and thus increasing the complexity of the production facility.
Modern vaccines such as protein subunits and VLPs use well-characterized antigens and thus offer a safety advantage compared to traditional inactivated or live-attenuated vaccines. Process development can be rapid, if the antigen is defined and there are a variety of expression systems from which to choose (e.g., bacterial, yeast, mammalian cells, insect cells). Efficacy is typically high with the help of an appropriate adjuvant, and the side effects can be minimized. The cost of goods is generally comparable to traditional vaccines, but lower than mRNA vaccines. Facility complexity is reduced, and the approach offers more flexibility and shorter production time.
In terms of vaccine development speed, nucleic acid-based vaccines are the fastest. As an established platform technology, mRNA also enables a tremendous amount of manufacturing flexibility and production speed. In routine, large-scale manufacturing, mRNA vaccines would require the least costly capital installation to produce the same amount of target volume. With the same scale, mRNA technology can be used to easily produce many more doses compared to that of an inactivated vaccine production line. These vaccines also require the least facility utilization, thus leaving room for multi-vaccine production. mRNA is nevertheless a new modality, and evolving regulatory guidelines can create uncertainty.
The scale of production and facility utilization also impacts the cost structure of vaccine manufacturing as illustrated by the cost model scenario simulation (Figure 6A). There were dramatic differences in the cost of production across Phases 1, 2, 3, and commercial scale for the different vaccine modalities. In Phase 1, small doses are produced using expensive equipment and as such, most costs are with fixed capital charges. As production is scaled, however, material and consumables costs became drastically higher (Figure 6B). In real-life situations, clinical materials are often produced in multi-product facilities or outsourced, thus sharing and reducing the absolute cost of capital and labor.
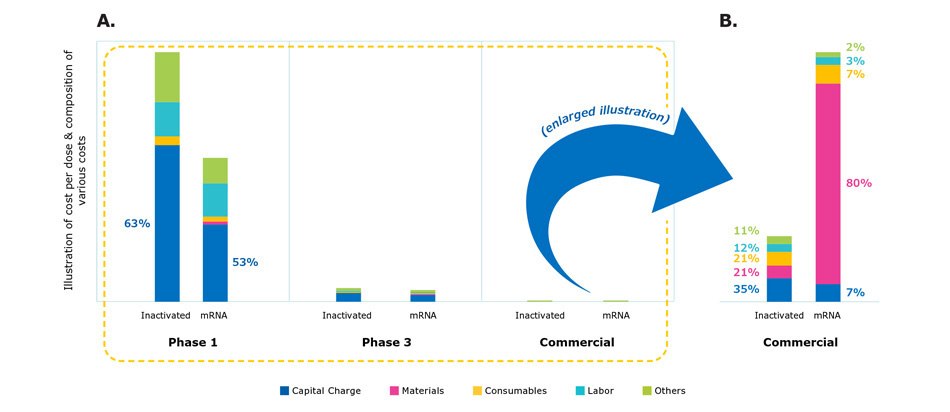
Figure 6.Comparison of cost structure in different manufacturing scales from clinical development to manufacturing.
Cost Modeling Provides Critical Decision-Making Insights for Optimizing Production Processes
The vaccine industry continues to grow and evolve. The speed at which vaccines were developed against SARS-CoV-2 and their success protecting against infection and mitigating symptoms reinforced their value and potential against a wide range of infectious diseases, cancer, and other conditions. It also inspired the pursuit of approaches to further accelerate the production of safe and effective vaccines. As a result, efforts are ongoing around the world to improve the efficiency of production with various global organizations, local governments, and biopharmaceutical companies launching initiatives centered on mRNA technology.
As the industry seeks to accelerate workflows, intensify production, and apply more flexible approaches to vaccine manufacturing, cost modeling can be a powerful tool for understanding and optimizing processes.
Combined with a qualitative and quantitative assessment of the production parameters associated with different modalities, the cost model described in this article can offer important insights for determining which vaccine type is best suited for a particular application. For example, this cost model revealed some key findings about mRNA vaccine production which indicates that this modality can be a robust starting point for production with low risk:
- Despite the overall cost of mRNA vaccine production being the highest among the different modalities, the platform technology and flexibility of these vaccines require the least capital investment when manufactured using single-use equipment.
- The smaller production scale reduces the complexity of facility design.
- A lower utilization rate means that more doses can be produced per batch.
Ultimately, selection of which vaccine modality will be produced requires cost considerations along with an assessment of available resources. In addition to applying a robust cost model, partnering with a technology provider with global experience in manufacturing all different types of vaccine modalities can further ensure a cost-effective, high-quality process.
Related Videos
Vaccine Development and Manufacturing Capabilities
We can help with cutting edge technologies, products and services for all your modalities from upstream to final fill.
Flexible Manufacturing
Use fexible manufacturing stategies can help meet growing vaccine demand.
To continue reading please sign in or create an account.
Don't Have An Account?